Cosmic explosions
Astrophysical transients are important for almost every aspect of astronomy. Transients are sources whose brightnesses are quickly changing, observable within a human lifetime, varying within seconds up to years.

DARK uses telescopes around the world and in space to study exploding transients, such as supernovae, gamma ray bursts, and tidal disruption events. They analyze data to understand the formation of stars, galaxies, and the universe as a whole.
The research at DARK also focuses on studying the properties and interactions of cosmic dust in galaxies. They work with other international scientists on projects such as STARGATE, the Young Supernova Experiment, and the Vera Rubin telescope Legacy Survey of Space and Time.
We use ground based telescopes around the world including La Palma, Chile, and Hawaii, as well as space based telescopes such as the Hubble Space Telescope, and the JWST to obtain photometric and spectroscopic transient data.
Analyzing these data with modern methods, we can understand how and why these phenomena occur, but also gain pivotal insight into many important topics in astrophysics, including the evolution of stars, galaxies and the entire Universe.
To fully explore the exploding transient universe and find answers to some of its outstanding questions, scientists at NBI are working together with other scientists from around the world on a number of projects. They are key members of several current and upcoming transient collaborations and surveys, such as STARGATE, the Nordic Optical Telescope GRB program, ENGRAVE - Electromagnetic counterparts of gravitational wave sources at the Very Large Telescope, SN2 or the Public European Southern Observatory Spectroscopic Survey for Transient Objects and the Mirror-slicer Array for Astronomical Transients (MAAT) collaboration.
Beside these, one of our gold-mines for transient data is the Young Supernova Experiment (YSE). It includes more than 100 astronomers from Denmark, the USA and the UK. Using the Pan-STARRS telescopes at the Mauna Kea Observatory in Hawaii to observe. YSE is designed to find transients within hours of their explosion, which provides invaluable information about the stars final evolution and explosive aftermath.
YSE is an international precursor transient survey to Vera C. Rubin Observatory Legacy Survey of Space and Time (LSST), which is predicted to stream more than a hundred thousand supernova events in just a single night, amounting to millions of supernovae over its 10 year timescale. To fully exploit its potential for supernova science, spectroscopic follow-up facilities are in high demand. DARK scientists are currently refurbishing and upgrading the Danish 1.54m telescope. The DK1.54m telescope is owned and operated by NBI and located at the European Southern Observatory at La Silla in Chile, just a stone’s throw away from the Vera C. Rubin Observatory.
To fully explore the exploding transient universe and find answers to some of its outstanding questions, scientists at NBI are working together with other scientists from around the world on a number of projects. They are key members of several current and upcoming transient collaborations and surveys, such as STARGATE, the Nordic Optical Telescope GRB program, ENGRAVE - Electromagnetic counterparts of gravitational wave sources at the Very Large Telescope, SN2 or the Public European Southern Observatory Spectroscopic Survey for Transient Objects and the Mirror-slicer Array for Astronomical Transients (MAAT) collaboration.
Beside these, one of our gold-mines for transient data is the Young Supernova Experiment (YSE). It includes more than 100 astronomers from Denmark, the USA and the UK. Using the Pan-STARRS telescopes at the Mauna Kea Observatory in Hawaii to observe. YSE is designed to find transients within hours of their explosion, which provides invaluable information about the stars final evolution and explosive aftermath.
- https://ui.adsabs.harvard.edu/abs/2021ApJ...908..143J/abstract
- https://ui.adsabs.harvard.edu/abs/2022arXiv221107128A/abstract
Aidan Sedgewick, Diego Farias, Luca Izzo, Charlotte Angus, Nandita Khetan, Christa Gall, Jens Hjorth, Radek Wojtak, Sandra Raimundo
The most common exploding transients are amongst the most luminous and are termed supernovae. Depending upon the properties of the stars and their evolution leading up to their explosion, many different types of supernovae can be observed.
Looking at stars which are less than about 8 times the mass of the Sun, they do not explode when they die. Instead they become compact objects known as white dwarfs. These are balls of carbon and oxygen, about the same mass as the Sun but squashed down into a sphere about the size of the Earth. They are safe from further gravitational collapse, but only if the mass of the white dwarf is not larger than about 1.4 times the mass of the sun.
In the event that the white dwarf has a companion star, it may steal material from it and increase its mass beyond the 1.4 solar masses limit. This can trigger fusion of the white dwarf material, leading to a thermonuclear explosion that eventually is so powerful that it disrupts the white dwarf entirely. The result is a very bright supernova known as a ‘Type Ia’ supernova.
Since the mass of the white dwarf is always very similar, these explosions appear to be rather uniform. Because of this, Type Ia supernovae can be ‘standardized’ and used to measure distances to the galaxies in which these exploding transients occur. Accurate distances are important to infer cosmological parameters, such as the Hubble constant.
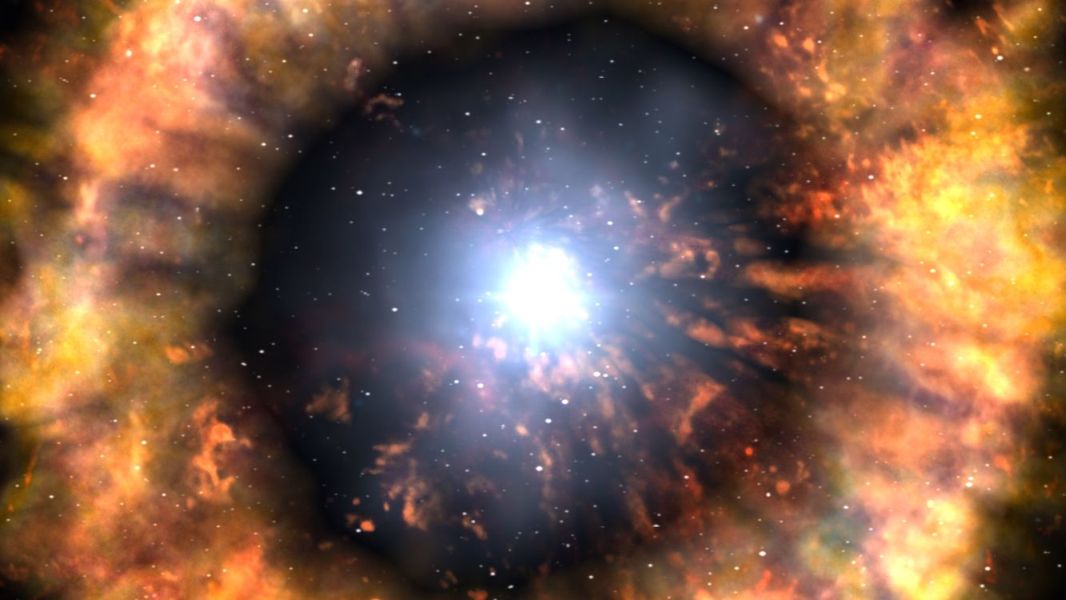
As part of the Young Supernova Experiment we are currently involved in research on supernova physics with a particular focus on catching the objects early using the Pan-STARRS telescopes on Hawaii to better understand the supernova process.
At DARK we are involved with follow-up observations of YSE targets using the Nordic Optical Telescope on La Palma, Wide Field Spectrograph (WiFeS) at Siding Spring Observatory, along with other observation programs outside of the YSE collaboration for example with X-shooter at the VLT. Research at DARK involves investigating both interacting supernovae (IIn, Ibn, Icn, Ia-CSM) and superluminous supernovae.
Interacting supernovae are core-collapse or thermonuclear supernovae interacting with a circumstellar material either rich in hydrogen, helium and rarely carbon/oxygen producing spectra with characteristic narrow emission lines.
The research currently being done at DARK revolves around the rate and underlying physics of hydrogen-rich interacting supernovae called type IIn as well as more exotic interacting objects like the helium-rich (type Ibn) SN 2021foa and SN 2022ihx. In particular, the former also contains an unusually large amount of hydrogen, suggesting a clear transition between Type IIn and Ibn, only observed in a handful of objects until today.
Diego Farias, Cecilie Cold, Charlotte Angus, Luca Izzo, Aidan Sedgewick, Christa Gall, Jens Hjorth
Stars that are less than about 8 times the mass of the Sun will not end their lives with a catastrophic explosion, but they will reduce to compact objects, after losing their external envelope of hydrogen, known as white dwarfs. These are balls composed of carbon and oxygen, with a mass similar to the Sun, but squashed down into a sphere about the size of the earth.
These compact objects are safe from further gravitational collapse, but only if the mass of the white dwarf is not larger than about 1.4 times the mass of the Sun. Otherwise Carbon ignition will lead to the complete disruption of the star and the consequent appearance of a type Ia supernova.
In the case that a white dwarf has a companion star, it may steal material from it, and during this matter accretion process, non-terminal thermonuclear explosions can repeatedly occur on the surface of the white dwarf, producing low-luminosity transient phenomena known as Classical Novae. In these events, newly-synthesized light elements such as lithium, are ejected and will enrich the interstellar medium with these elements.
Lithium, in particular, is a peculiar element, being the only metal formed during the Big Bang nucleosynthesis. However, observations of some young stars have shown that their abundance of lithium is larger than the amount of lithium produced in the Big Bang, suggesting the existence of active factories of this element in the Universe.
At NBI, scientists are actively involved in addressing challenges about the physical mechanisms of Novae and, in particular, their role as the main factories of lithium in our Galaxy, and in nearby systems. Particular emphasis is also placed on elucidating the origin of the high-energy emission observed in Novae.
Until recently it was believed that this stems from nuclear reactions happening during the repeated nova explosions. However, new observations by the Fermi-LAT detector have confirmed that shocks produced by colliding shells of previously ejected material can accelerate particles, causing the observed high-energy emission.
- https://ui.adsabs.harvard.edu/abs/2022MNRAS.510.5302I/abstract
- https://ui.adsabs.harvard.edu/abs/2020A%26ARv..28....3D/abstract
- https://ui.adsabs.harvard.edu/abs/2020MNRAS.492.4975M/abstract
- https://ui.adsabs.harvard.edu/abs/2020NatAs...4..776A/abstract
Luca Izzo
The most massive stars in the Universe have masses between ~20 and ~40 times the mass of the Sun. These stars end as core-collapse supernovae but their lives and deaths are very extreme. Such stars can throw off large amounts of their outer hydrogen and helium layers in massive eruptions all at once that may mimic a real supernova.
When the fuel of these stars runs out, their cores immediately collapse into a black hole, while the surrounding dense matter forms an accretion disc around the black hole which feeds it. This process produces jets of very energetic radiation, which quickly escape from the progenitor stars at relativistic velocities.
The interaction of the jet with the stellar remnant gives rise to a cocoon component, which expands laterally to the jet, and that can be detected in the X-rays and optical as rapidly decaying emission. Moreover, once the jet breaks-out from the progenitor star, it gives rise to a burst of gamma rays that will be the first signature of the death of a very massive star, if the jet points along our line of sight.
We call these events gamma ray bursts, and they are accompanied by a special type of core-collapse supernova, characterized by very large expanding velocities and kinetic energies.
Typically, gamma ray bursts are so bright that they can be seen out to very large cosmic distances. This allows us to study some of the stars born in the very earliest stages of the Universe, and at the same time to study the properties of the immediate environments, and their galaxies, at very high redshifts.
Recently, NBI researchers discovered such a type of supernova that turned out to be a gamma ray burst, but with an off-axis jet of gamma rays that was not pointing towards the earth, and hence remained undetected. These "orphan" GRBs represent one of the main targets of incoming deep optical surveys, such as the Vera Rubin telescope, which is going to start to get data in the next few years.
- https://ui.adsabs.harvard.edu/abs/2023arXiv230207891M/abstract
- https://ui.adsabs.harvard.edu/abs/2022A%26A...659A..39M/abstract
- https://ui.adsabs.harvard.edu/abs/2020A%26A...639L..11I/abstract
- https://ui.adsabs.harvard.edu/abs/2019Natur.565..324I/abstract
Luca Izzo, Jens Hjorth
Kilonovae are electromagnetic counterparts to mergers of binary neutron stars, signalling the formation of heavy elements through neutron capture. Kilonovae have now been found in gravitational wave events and in short and long gamma-ray bursts.
The ongoing O4 observing run with the gravitational wave detectors LIGO, Virgo and KAGRA is expected to lead to new discoveries of binary neutron star mergers which we will follow up with a range of observing facilities, including VLT, ALMA and JWST as part of the ENGRAVE collaboration. We will also observe kilonovae spectroscopically with the MAAT instrument which is being developed.
Jens Hjorth, Luca Izzo, Aprajita Hajela, Christa Gall, Nandita Khetan
Superluminous supernovae (SLSNe) are a rare class of highly luminous transients with peak magnitudes a factor of 2 or more higher than a classical core collapse supernova. They have been detected up to redshift 4 and would be detected to even higher redshifts (z>10) with advanced telescopes like JWST, Roman and Rubin.
As such, they offer an appealing tool to study multiple areas of stellar and galactic evolution like physics behind their enormous energies, probing their host proto-galaxies, trace star formation at high redshifts, etc. Additionally, their detectability at such high redshifts could also be used to exploit them as cosmological probes to trace the expansion history of the Universe.
SLSNe, like a supernova type Ia, have uniformity in their light curves and spectroscopic data which can be leveraged to standardise these events for measuring cosmological distances and constraining Dark energy equation of state.
With surveys like Dark Energy Survey, YSE, LSST, etc. we are involved in searching and characterising these explosive events for studying their properties, progenitor channels and explosion mechanism. Additionally, we are also involved in advancing the work towards using SLSNe as distance indicators. In particular, we explored high redshifts SLSNe (z >1) characterising their light in their rest frame Ultraviolet emission and determined correlations between their light curve properties.
These studies offer interesting insights into using them for cosmology as well as the nature of these peculiar objects, encouraging further exploration. With a robust framework already in place, we are ready to explore the wealth of SLSNe samples that will be provided by instruments like Rubin and JWST, and eventually with the Roman telescope.
Nandita Khetan, Charlotte Angus, Jens Hjorth
Most galaxies in the Universe host a central supermassive black hole (SMBH), weighing roughly 106 - 108 M☉. Most of these SMBH are 'quiescent' as they do not accrete gas as a rule. But every once in ~100,000 years, an unfortunate star comes too close to one of these central quiescent SMBH, and gets pulled apart by its strong gravitational pull, forming an accretion disk, as well as sometimes launching relativistic jets.
These are known as tidal disruption events (TDEs), and are a probe of the inner regions of the distant galaxies, whose centers remain otherwise unresolved with our current telescopes. The lifetime of a TDE is comparable to a human lifetime, which provides us with a unique opportunity to study accretion physics, and jet launching mechanisms from start to finish at the scales of these SMBHs.
At NBI, researchers follow-up these events across the electromagnetic spectrum, starting soon after the discovery until late-times. Even with the currently small sample of TDEs, we have observed a significant diversity in their observational signatures. Not every TDE has previously shown evidence of a prompt launch of a jet. However, a modest fraction of TDEs have recently been discovered with a delayed radio emission, indicating the presence of an off-axis jet, or an entirely new phenomenon that hasn’t been previously studied/observed in TDEs, putting these subset of TDEs at the forefront of our research focus.
Another area of peak research interest is the low mass end of the SMBH (< 106 M☉), also called intermediate mass black holes, or IMBH. They are of particular interest as they hold the potential to provide unique insight into the evolution of black holes from the stellar mass to supermassive scales.
Researchers at NBI have led a multiwavelength study that provided strong evidence of one such tidal disruption, AT 2020neh, of a star by an IMBH, providing a galaxy-independent method of measuring the masses of intermediate-mass BHs.
The field of TDEs is still new, but rapidly gaining momentum as we arrive into the golden age of TDEs, with the state-of-the-art optical sky surveys, one of which is YSE, that many researchers at NBI are a part of. Rubin/LSST is expected to discover potentially tens of thousands of TDEs during its lifetime. With proper multiwavelength follow-up programs, we will be able to tap into the potential of this new and upcoming field.
Aprajita Hajela, Charlotte Angus
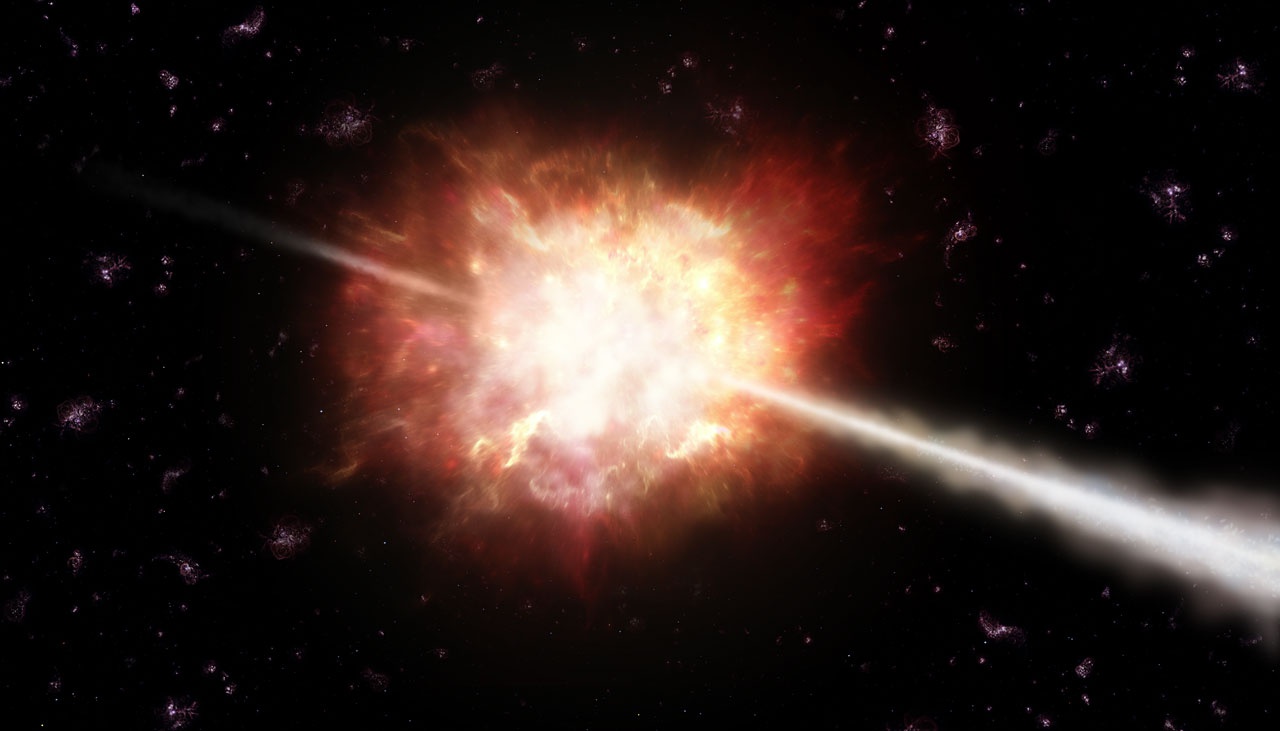
Events like Gamma Ray Bursts and TDEs have shown to launch extremely energetic, highly collimated jets. The interaction of these jets with the surrounding medium are one of the predominant sources of emission in these events, and provide key insights into the ambient medium of these catastrophic events.
Jets themselves only last for ~ a few seconds, but they drive a relativistic shockwave in the medium they are traveling in. The shock accelerates the population of electrons in a power-law distribution, and amplifies the magnetic field. These electrons gyrate in the magnetic field and cool down via synchrotron radiation. This is known as the jet afterglow that we observe across the electromagnetic spectrum, for ~10s of years after the explosion.
Events that launch a jet aligning with our line of sight are extremely bright, and hence detected promptly in γ-rays. However, since these are strongly Doppler-beamed sources, we miss a large fraction if they are not pointing directly at us.
The shockwave producing the jet afterglow decelerates with time, and becomes more expansive. Following up the resulting jet afterglow across the spectrum help us identify the spectral features (for e.g. breaks in the spectrum) of the synchrotron spectrum that allow us to tightly constrain the density of the ambient medium, the total energy budget of the event, as well as the strength of magnetic field, and the shock microphysics.
All of these are not properly understood in the transient field. Also, it is not understood why only a subset of GRBs and TDEs produce these relativistic jets. The study of jet afterglow, therefore, allows us to improve our understanding of the physical mechanism behind these energetic events, and why they are only unique to a subset of these broad classes of explosions.
Aprajita Hajela
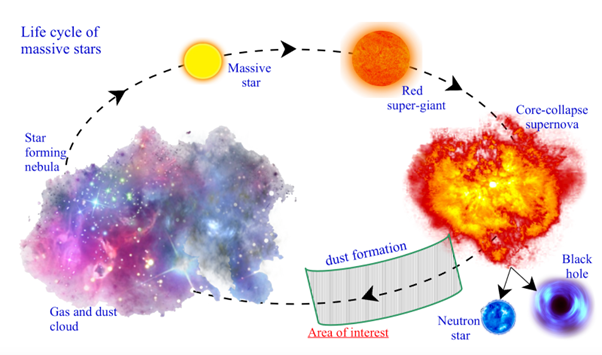
Cosmic dust has a profound effect on the evolution and spectral appearance of galaxies. Large amounts of cosmic dust have been observed in galaxies throughout the universe, but their origin and evolution remains a mystery. Core collapse supernovae are the prime factories in the universe of elements such as carbon, oxygen and silicon and importantly thought to be efficient producers of dust.
At DARK, we are working on a series of exciting theoretical and observational projects that aim to quantify the role of supernovae as dust producers. Our ongoing project, on detecting extragalactic supernovae and analyzing the photometric and spectroscopic data of mid-IR wavelengths using JWST, is complemented by our modeling efforts on the detailed chemistry of winds or ejecta in various types of supernovae.
We recently discovered the second-largest mass of dust present in extragalactic supernovae (SN 2004et) ever detected. We found that large, micron-sized dust grains are formed rapidly, shortly within tens of days after explosion in some core collapse supernovae, followed by accelerated dust production over a period of up to 25 years.
Our models explore the complex shock-dust landscape in astrophysical environments that governs the evolution of different objects such as the interacting supernovae, classical novae, common envelope stars, interacting binaries, and also the winds around AGNs.
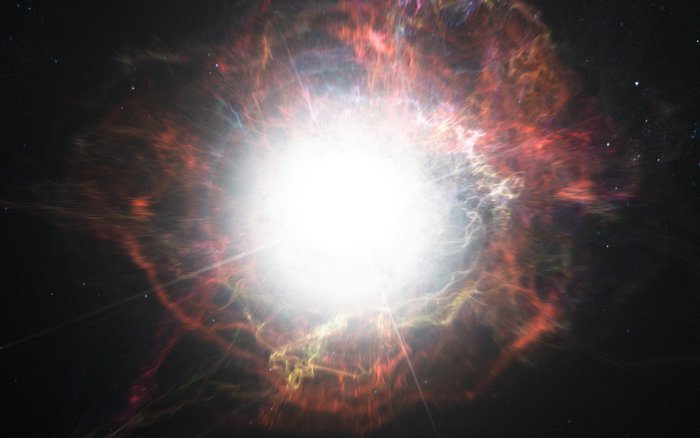
- https://ui.adsabs.harvard.edu/abs/2014Natur.511..326G/abstract
- https://ui.adsabs.harvard.edu/abs/2023arXiv230110778S/abstract
- https://ui.adsabs.harvard.edu/abs/2022A%26A...668A..57S/abstract
- https://ui.adsabs.harvard.edu/abs/2022ApJ...933...89S/abstract
- https://ui.adsabs.harvard.edu/abs/2018ApJ...868...62G/abstract
Arka Sarangi, Christa Gall, Jens Hjorth
Recently, several lensed supernovae have been discovered and LSST and Roman are expected to detect hundreds. With lensed transients we will determine time delays for Hubble constant measurement, obtain spectra of distant, magnified supernovae in unprecedented detail, investigate the properties of their host galaxies, and perhaps elucidate the progenitors of supernovae.
We are currently pursuing this with JWST, HST and VLT, partly through involvement in the LensWatch collaboration, and will expand this with MAAT observations.
- https://ui.adsabs.harvard.edu/abs/2023ApJ...948..115P/abstract
- https://ui.adsabs.harvard.edu/abs/2019MNRAS.487.3342W/abstract
- https://ui.adsabs.harvard.edu/abs/2019MNRAS.487.3342W/abstract
Jens Hjorth, Radek Wojtak, Christa Gall